10:30 am - 11:00 am CDT
E1: AI for Improving System Performance
System Optimization
Artificial Intelligence: The Newest Tool in the Building Performance-Based Design Toolkit
Artificial Intelligence has been heralded as the miracle technology of our time and is expected to help solve the world's problems ranging from climate change to dandruff. While some of the claims made about AI may be unrealistic or too optimistic, when applied in the right context and to the right types of problems it can be a useful tool. As we grapple with the impacts of climate change and new demands being placed on building systems, AI can be applied, along with the more traditional repertoire of Building Performance-Based Design Tools, to help propel design performance to new levels.
This presentation will explore the suite of tools available to provide design guidance for lab buildings and the strengths and weaknesses of AI compared to more traditional tools, such as wind-tunnel testing and Computational Fluid Dynamics. Examples where AI is currently being applied to address conventional design challenges and explore the impacts of a changing climate will also be discussed. Finally, we will examine the potential barriers to adoption as well as steps to expand the use of AI to tackle a wider scope of design problems.
Big Data: Evolution of Design + Operations
Advanced, energy-efficient, and decarbonized lab facilities face a growing problem, maintaining and operating the complex systems behind them. Translating engineered efficiency into real-world operations can be a challenge with the ever-growing lack of skilled workers to maintain operations.
In almost every corner of the modern world, the technology we interface with collects and uses data to personalize and optimize our experiences. The data trends collected speed up our online shopping experiences, tailor the way we consume information, and allow us to navigate the world faster and more efficiently. It is therefore no surprise that modern buildings produce vast amounts of data. By properly harnessing that data, facility data can prove to be an important utility in simplifying and maintaining energy-efficient operations in an ever more complex built environment.
Artificial Intelligence, Machine Learning, and Their Impact on Sustainability in the Lab
Artificial Intelligence (AI), along with Machine Learning (ML), promises to play a significant role in the way science and technology research environments are planned, constructed and operated in the near and distant future. The results will greatly enhanced discovery and science related investigations producing facilities destined to impact human health in a positive way. In addition, AI and ML will assist architects, engineers and builders identify ways to maximize resource conservation and sustainability once the facility is in operation. This will not doubt have extensive influence in the amount of energy and resources consumed by research buildings, and other technically advanced structures.
Though AI does offer numerous benefits, it is not without its challenges, risks, and uncertain issues to consider. Continuous human oversight, data influence and ethical concerns are just a few of the elements that must be evaluated when integrating AI and ML into a building’s sustainability approach. In addition, AI should be examined as an instrument to enhance, rather than replace, the skill and expertise of facility owners, managers, and engineers, who play an integral role in a building's operations.
This presentation will explore and discuss several of the salient aspects of artificial intelligence and machine learning, and how they are predicted to affect the way facilities address sustainability and resource conservation in an effective and efficient way.
E2: Purchasing Equipment for Better Results
Green Labs
Industry Ecolabel Efforts to Drive Scope 3 Emissions Down
Scope 3 emissions in the biotech and pharma industry remain 4.6-fold higher than Scope 1 and Scope 2 combined, as demonstrated in the 2023 Carbon Impact of Biotech & Pharma Report from My Green Lab. The vast majority of these Scope 3 emissions come from purchased goods and services, highlighting the impact of understanding and reducing Scope 3 emissions through metrics, manufacturer transparency, product design, and better procurement decisions.
To support this aim, manufacturers, distributors, sustainability and LCA experts, procurement professionals, academics, and auditors from throughout the industry recently completed a stakeholder consensus process facilitated by My Green Lab that drove improvements in the ACT Ecolabel for life sciences equipment, reagents, and consumables.
In this talk, learn about the goals of these changes, why independent third-party verified information is critical for effective sustainability purchasing policies, and how to integrate this information into procurement. Discover how the various environmental impacts of life sciences products are weighted by the U.S. EPA and ACT Label for a true and transparent understanding of product sustainability. Attendees will also learn about new legislation that requires third-party verification of sustainability metrics and marketing claims, and manufacturers will learn how to create better products and reportable sustainability outcomes through specific changes in product design and production.
Any Chance to Reduce Your Power Consumption in the Lab?
The impact of power consumption in the laboratory is neither limited to the very small instruments nor to the building air condition system. Labs need to mix and to spin, to heat and to cool, to transfer, and to measure, scientists depend on instruments. Nearly all instruments require electric power.
But how much power do these instruments really need? Everyone is aware of the energy-guzzling ULT freezers, but they are not the worst ones as quite often announced. Especially the broad range of different und multiple mid-sized lab equipment has a far bigger impact than you may expect.
Power consumption measurements require standardized conditions and repetitions.
Learn about power consumption data from different product groups and indications if and how power can be saved.
The carbon footprint analysis of complete instruments is still a future topic, but the carbon footprint of usage can be calculated. The supplier’s Scope 3 emissions are your Scope 2 emissions.
Eppendorf is assessing the current status and reviewing the best options for power savings in order to make them a reality. We want to give some insights what are the challenges on the manufacturer side and how far science can be pushed to the “green area” when saving power in the laboratory.
Cheap or Efficient? Aligning Incentives for Better Lab Equipment Purchases
In the early days of focus on laboratory sustainability, ultra-low temperature (ULT) freezers were identified as one of the most energy-intensive pieces of research equipment. ULT numbers were also growing rapidly with increased federal support for biomedical research. Several organizations, including Labs21 (I2SL’s precursor), supported initiatives to address this growing driver of high energy use in lab buildings.
In 2009, Stanford University was one of the first higher education institutions to use a self-funded rebate program to encourage researchers to purchase more energy efficient ULT models. Fifteen years and hundreds of purchases later, Stanford’s lab freezer rebate program has evolved in response to market changes and technology innovations. This presentation will recount the origins of the program, disclose some of its successes and shortcomings, and share ideas for a more sustainable approach to biological sample storage.
E3: Reducing Carbon in Commercial Labs
Sustainable Design
A Paradigm of Sustainability in Pharmaceutical Packaging Facilities
This facility is powered entirely by electricity, achieving zero operational carbon emissions. Onsite ground-mounted solar photovoltaic systems contribute to the facility's energy needs, ensuring a clean and renewable power source. Embodied carbon modeling was prioritized during construction, minimizing the carbon footprint associated with materials and processes.
The facility boasts significant achievements in water conservation, implementing advanced technologies to reduce water consumption. Waste reduction initiatives are paramount, employing innovative strategies to minimize environmental impact. This commitment to sustainability is underscored by the facility's LEED Gold certification, recognizing excellence in green building practices. Additionally, the facility prioritizes employee well-being by obtaining Fitwel 2-star health and wellness certification, creating a healthy and productive workspace.
In summary, this pharmaceutical packaging facility stands as a beacon of sustainability, incorporating cutting-edge technologies and conscientious practices from its inception. By seamlessly blending environmental responsibility with operational efficiency, the facility serves as a model for sustainable industrial development in the pharmaceutical sector.
Template for a Net Zero Mindset
Establishing net zero as an ultimate goal for lab projects is becoming more commonplace in both the public and private sectors. Current reality for many owners and institutions is that the goal is not achievable in the near term. Alternatively, a series of steps may be required to first define what “net zero” means for an individual project, and then to develop an implementation plan to achieve results incrementally. Key to the process is the adoption of a net zero mindset--strategizing major infrastructure moves to align with steps towards net zero. Starting with the owner perspective, what are the steps to establishing a net zero mindset for a new commercial lab building? And what are the key issues to consider for engaging the entire design team through the process? The planned new net zero campus for Cell Signaling Technology (CST) will be introduced as a case study to demonstrate the process from vision to implementation. The design sets a new benchmark for transforming an environmentally depleted site into a climate-friendly campus. Building electrification and onsite renewables address CST’s Climate Goal. Heating, cooling, and hot water for the building will be sourced from geothermal well fields. Additional energy conservation and embodied carbon reduction measures include maximizing onsite solar PV, battery storage, high efficiency heat recovery, shared fume hood strategies, innovative high-performance curtainwall, and mass timber construction for non-lab areas.
Design Approaches for Decarbonization in Core and Shell Commercial Science Buildings
As the urgency to combat climate change grows, the built environment plays a critical role in achieving global decarbonization goals. Core and shell commercial science buildings, which serve as the foundation for life science and other laboratory type tenant fit-outs, represent a significant opportunity for implementing sustainable design strategies. In this presentation, we will explore innovative approaches to reduce carbon emissions in core and shell buildings while maintaining functionality, occupant comfort, and economic viability. Key strategies discussed will include understanding core and shell buildings, decarbonization techniques, passive and active design technologies and the review of real-world case studies.
By prioritizing decarbonization strategies in core and shell commercial science buildings, we can create sustainable, resilient, and future-proof structures that contribute to a low-carbon future. Let's explore how design innovation can lead the way toward a more environmentally responsible built environment.
E4: Advanced Energy Sources
Decarbonization
Advanced New Solutions in Laboratory Geo-Exchange Systems Design
As research companies grapple with providing laboratory services at reduced environmental impacts, they’ve traditionally been forced to respond with ever more intransigently specific and often costly design solutions. The developers and designers of the state-of-the-art Ridgeway Science and Technology building in Boulder, Colorado, are looking to buck this trend with their innovative new take on laboratory-based geo-exchange systems. A clever combination of advanced engineering design and systems integration including coaxial boreholes and concentric bore field design linked to a photovoltaic thermal system transforms the standard geothermal approach into a win/win design, which saves on construction first costs, improves upon building energy efficiency and payback, and provides a new level of flexibility for a variety of changing laboratory needs. The system incorporates hot and cold Borehole Thermal Energy Storage (BTES) fields. Not only is the Ridgeway GeoSolar system integral to the facility’s Net Zero Energy, Net Zero Carbon and LEED Platinum design strategy, but the design concept also aims to offer similar advantages as a new model for other laboratory developments to follow.
The Carbon Conundrum of Back-up Power
As we work toward decarbonizing our lab buildings, fossil fuel combustion is being phased out of HVAC, water heating, and process applications, but what do we do about back-up power?
Emergency power systems are critical for maintaining essential services during outages, but traditional generators still rely on diesel or natural gas. Battery storage systems are often identified as a decarbonized option, but these systems can be prohibitively expensive and require large areas if extended run-time is required. Beyond the cost and space considerations, batteries themselves are very high in embodied carbon, so from a whole-life carbon standpoint, is a battery solution better?
This session compares traditional diesel generators to fuel cell, renewable diesel, and lithium batteries in different operating scenarios to evaluate whole life carbon impacts. Beyond the initial comparison and tipping points that drive carbon intensity for different options, we explore how to think differently about power systems to find an optimized solution that provides the required emergency power but also provides additional operational benefits to the system.
Integrating Renewable Energy Into Laboratory Design
The renewed focus on global warming and climate change has prompted the life science sector to reevaluate the design and engineering of its facilities. Laboratories are among the largest sources of greenhouse gas emissions because of their high energy consumption. By using renewable energy sources, laboratories can reduce their environmental footprint and reliance on conventional energy sources while lowering their carbon emissions. While the initial investment in renewable energy can be expensive, available incentives and long-term savings in operational expenses can offset upfront costs.
This presentation explores the various types of renewable energy systems, what factors to consider when choosing them, and how they can benefit a laboratory facility. The speaker will explain the advantages, potential incentives, and challenges of integrating renewable energy systems into laboratory facilities and how they can improve corporate social responsibility and the institution’s reputation.
Thank you to our sponsors!
Platinum

Gold
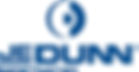
Silver
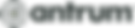

Bronze

